​
The reacting flow and numerical methods group looks into the numerical simulation of turbulent reacting flows and multiphase flow (e.g. spray injection, flash boiling flow) , with a focus on the development of moment-based models (e.g. Conditional Source Term-Estimation) and novel computational methods for post-processing (e.g. CEMA and SPDMD). The fundamental nature of the research entails that the group can easily transfer the knowledge and skills to a wide range of engineering applications including but not limited to combustion engines (IC and aerospace), burner design, hydrogen safety, etc.
Model development for numerical simulation of turbulent reacting flows

The long-term goal is to develop more reliable Computational Fluid Dynamics (CFD) approaches that can simulate a very wide range of propulsion systems, including those systems operating under extreme conditions with alternative fuels. In this Discovery Grant Project, the focus will be given to the fundamental development of a moment-based model, i.e., Conditional Sourceterm Estimation (CSE), with a particular emphasis on its application for partially premixed flames, which later can be extended to other challenging conditions. Specific objectives of this proposal include:
-
To construct low-dimensional chemistry manifolds for various renewable fuels.
-
To develop and assess PDF models for various flame configurations.
-
To integrate findings from previous objectives in a CSE-based model focusing on the predictions of thermodynamic properties and emissions of partially premixed flames.
Hydrogen Center of Excellence Project on Active Flow Controller for Flashback Mitigation
0 % by vol. H2
Currently, there are immediate plans to introduce a 15% hydrogen blend with natural gas in distribution systems within the next few years in Alberta. While this represents a positive step towards reducing greenhouse gas emissions, the true potential for substantial reduction lies in higher hydrogen blends, exceeding 50%. Alberta's hydrogen roadmap plan published in 2021 has indeed suggested pure hydrogen networks and communities will be demonstrated for 200,000 residences within the next 5-10 years, taking advantage of expected continued growth in the Alberta housing market. However, the characteristics of hydrogen combustion introduce critical challenges with repercussions that directly impact end-users.​ One of the primary concerns is hydrogen flashback, wherein the hydrogen flame propagates upstream to the low-velocity region of the approaching premixed hydrogen-air flow. For this research, we look into the numerical models for turbulent hydrogen flames where the precursors of hydrogen flashback will be identified.
40 % by vol. H2
95 % by vol. H2
Detonation waves developed during the abnormal combustion can cause more substantial damage in an extremely short time which leaves practically no chance of containment or mitigation; therefore, it is vital to take every measure to stop a flame from accelerating and reaching a condition where it can transform to a detonation wave. Although extensive studies have been carried out by different groups focusing on Deflagration to Detonation Transition (DDT) development in open channels, most of these projects were not extended to enclosed spaces with extreme initial conditions. This project first intends to study the fundamentals of the DDT process in a confined space. Moreover, the project will help develop a practical and reliable modelling approach for simulating DDT at large scales through an in-depth analysis of the DDT mechanism with the view of obtaining more insight into possible alternatives for describing the transition mechanism through a model instead of fully resolving it. This research can then be directly targeted to the key societal challenges of climate change and air quality. The global nature of the proposed solution to accelerating the adoption of high-efficiency, near-zero emissions low-carbon transport further speaks to the importance of this work.
Numerical investigation of confined space deflagration-to-detonation transition
Understanding detonation waves interacting with obstacles is critical for the study of detonation wave propagation. Under proper conditions, when a detonation wave diffracts from an obstacle, it may first quench and then re-initiate. The purpose of this project is to numerically evaluate the effect of different effective activation energies on the detonation cellular dynamics for the hydrogen-air detonation attenuation problem. Specifically, we investigate how the cell size and structures change with an increasing effective energy for the chemical diffusive model. In addition, the behavior of detonation decoupling and re-initiation when a cellular detonation wave diffracts from a cylindrical obstacle is studied with emphasis on the role of effective activation energy on the critical outcomes of detonation re-initiation.
Numerical investigation of detonation attenuation and reinitiation
Translation of numerical methods for a zero-carbon future into industry
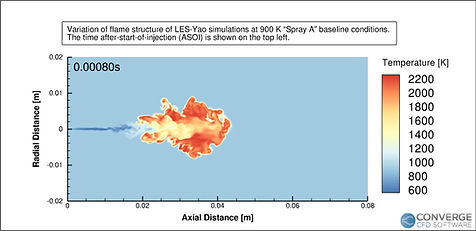

Among all alternative fuels, compressed or liquefied hydrogen (H2) and ammonia (NH3) have been recognised as promising choices owing to their zero-carbon nature. Much of today's industrial development currently relies on the use of computational fluid dynamics (CFD) software. However, today's software is mostly designed around fossil fuels, which can behave very differently from zero-carbon alternatives. This project then seeks to incorporate models developed at Oxford into one of the leading global (US in origin) CFD codes (Converge). If successful, this will lead to improved virtual world development for all of Converge's customers (which include much of the automotive and marine industry, as well as renewable energy providers). Knowledge of four underpinning research grants covering the fundamental characteristics of alternative fuels over the span of 10 years will be consolidated and translated into Converge's next software release for commercial development. A dedicated data platform will be created, enabling academia and industry to access our data for their needs. The timely nature of this project will make it a world-leading activity. This project collaborates closely with the Oxford Thermal Propulsion System Research Group (TPSRG).
The long-term goal of this research area is to develop more reliable Computational Fluid Dynamics (CFD) approaches that can simulate and diagnose the deflagration and detonation transition process in confined spaces, including those systems operating under extreme conditions with alternative fuels. For this project, the focus will be given to fundamental model development with a particular emphasis on hydrogen-air/oxygen mixtures DDT at laboratory conditions, which later can be extended to other challenging conditions. The key fundamental question this project aims to answer is:
-
What are the driving mechanisms for enclosed space deflagration to detonation transition for hydrogen-air/oxygen mixtures, and how are they different from other fuels?
The detailed objectives are as follows:
-
Numerical validation and study of DDT mechanism.
-
Novel post-processing technique for DDT analysis.
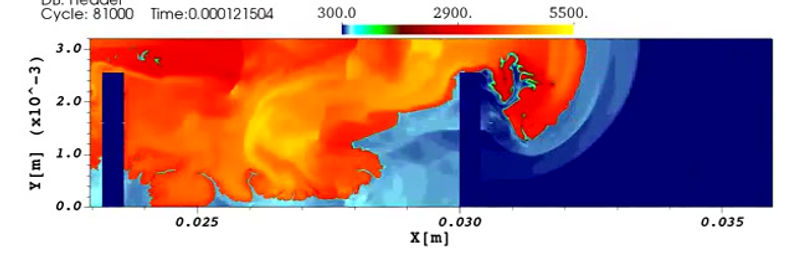
Computational study of deflagration to detonation transition for hydrogen safety
Research Sponsers
We gratefully acknowledge the generous support from our funding sources.
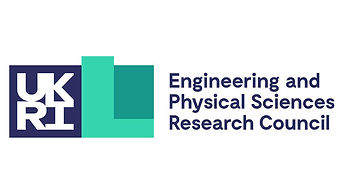

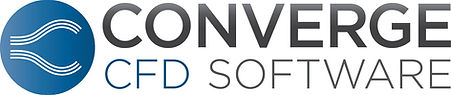


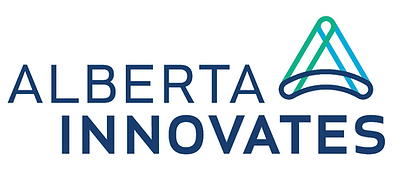
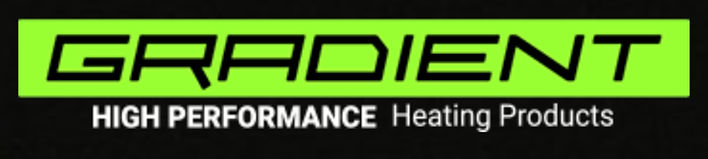
Research Collaborations
We also gratefully acknowledge our collaborators from various research institutes.
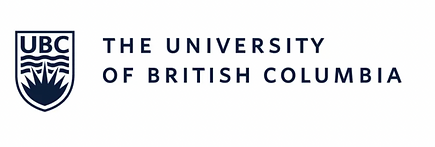
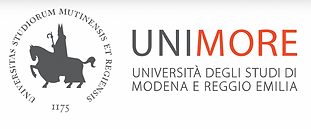
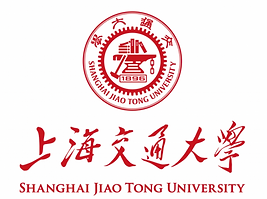
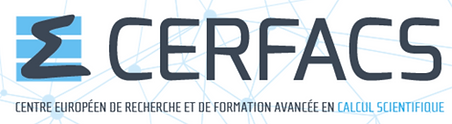
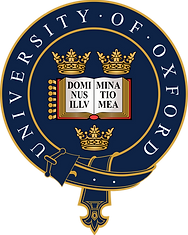

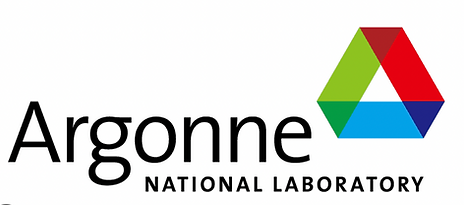

